An experiment recently conducted at CERN using its Compact Muon Solenoid (CMS) detector has led to new high-precision measurements involving a key component of the Standard Model of physics.
The results of the experiment, which relied on its CMS general-purpose detector at the Large Hadron Collider (LHC), were presented by the CMS collaboration at the annual Rencontres de Moriond conference and revealed the collaboration’s measurements of the effective leptonic electroweak mixing angle, resulting in what is recognized as the greatest precision ever attributed to measurements undertaken at a hadron collider facility.
CERN and the New Physics
In the past, discoveries at CERN have ranked among the most significant in modern physics. Results obtained during testing at CERN have led to a wealth of new information, allowing for predictions that help to check the theoretical models physicists rely on.
Advancements at CERN have also included the famous discovery of the Higgs boson in 2012, which physicists recognize as being potentially crucial in the search for evidence of dark matter.
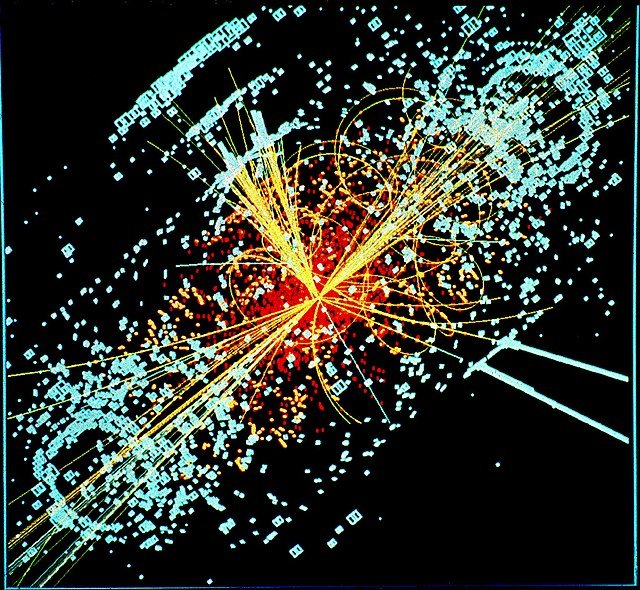
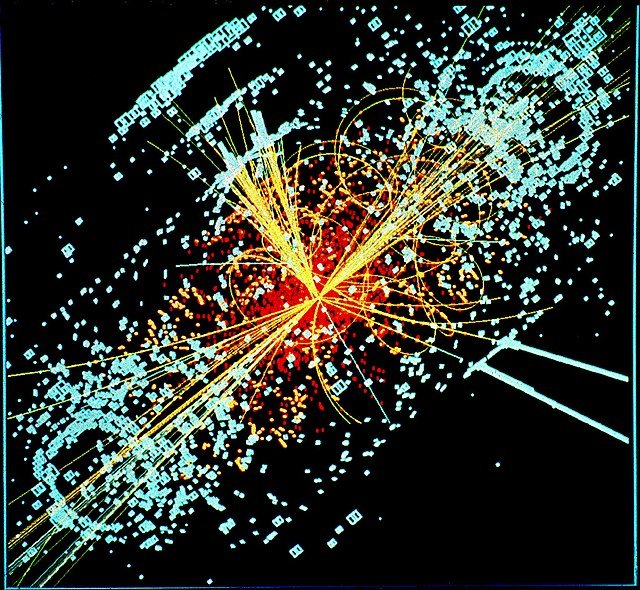
The CMS collaboration’s latest findings align with predictions derived from the Standard Model of particle physics, which presently describes three of the four known fundamental forces. It remains the most precise description of elementary particles and their interactions to date.
Through ongoing measurements and theoretical calculations, physicists hope to further refine the Standard Model, allowing even more precise predictions about phenomena in our universe that currently remain mysterious, comprising an area of study that scientists often call “new physics.”
One reason for this is that highly precise measurements of particles characterized by the Standard Model allow undiscovered phenomena to reveal themselves as discrepancies that appear between our calculations and the actual quantities measured during experiments at facilities like CERN.
The Electroweak Mixing Angle
A fundamental parameter in the Standard Model of particle physics, the electroweak mixing angle (also known as the Weinberg angle) is what unifies the electromagnetic force and the weak force into a single electroweak force.
The electroweak mixing angle is significant because it conveys how two seemingly very different forces represent different aspects of a single, unified force at high energy levels. According to the Standard Model, symmetry exists between electromagnetic and weak forces at very high energies, as described by a group of gauge bosons, which are bosonic elementary particles that play the role of force carriers (i.e., particles that give rise to forces between other particles) for elementary fermions.
The electroweak mixing angle allows the measurement of how much the original gauge bosons mix, resulting in the formation of the photon and the Z boson, which along with the W boson was first discovered at CERN in 1983. The masses of the Z and W bosons are dependent on this mixing (whereas photons remain massless), and the value of the electroweak mixing angle is also what governs the strength of the weak force, which gives rise to the decay rates of various particles, as well as scattering cross-sections that physicists observe during particle physics experiments.
Significantly, the value of the electroweak mixing angle is not predicted by the Standard Model and must be calculated through experimentation. Hence, its precise measurement plays an important role in efforts to test the accuracy of the Standard Model, as well as the detection of “new physics” that hints at phenomena currently beyond our theoretical models.
Precision Measurements at CERN
In the past, during separate experiments conducted with the CERN LEP collider and the SLD experiment at the SLAC National Accelerator Laboratory– the two most precise measurements ever recorded involving the weak mixing angle at that time–different values were obtained, a result that perplexed the world of physics.
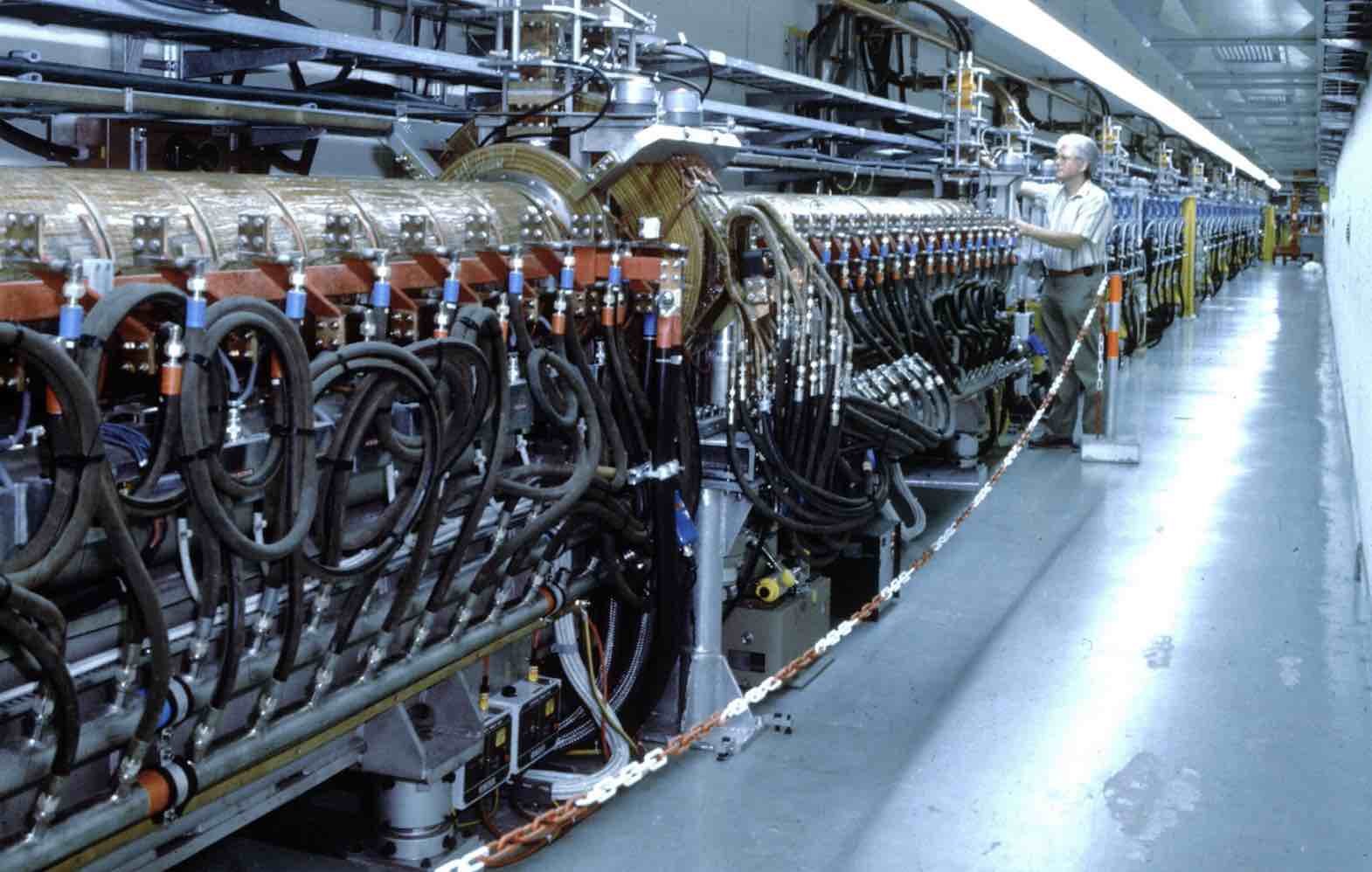
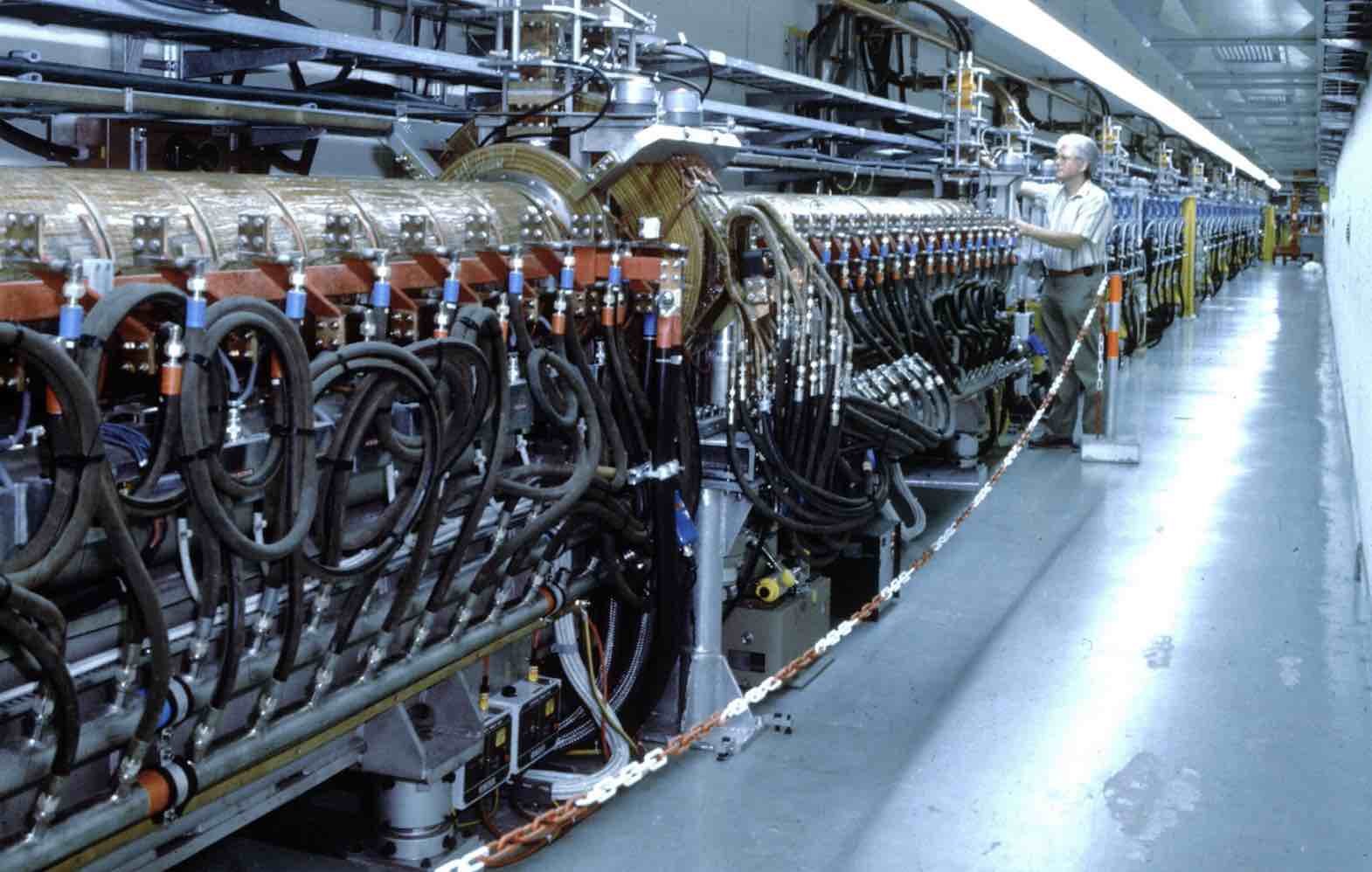
However, that discrepancy may now be close to a resolution since the latest measurements at CERN are a close match for predictions in the Standard Model.
Patricia McBride, a spokesperson with the CMS, said the new results are a clear indication of the level of precision that can be obtained during experiments with hadron colliders like the one at CERN.
“The analysis had to handle the challenging environment of LHC Run 2, with an average of 35 simultaneous proton-proton collisions,” McBride said in a statement.
“This paves the way for more precision physics at the High-Luminosity LHC, where five times more proton pairs will be colliding simultaneously,” McBride added.
The Most Precise Hadron Collider Measurement Yet
Experiments involving electron-positron collisions, such as those carried out at CERN with the Large Hadron Collider (LHC), are ideal for high-precision measurements that can gauge the parameters of the Standard Model.
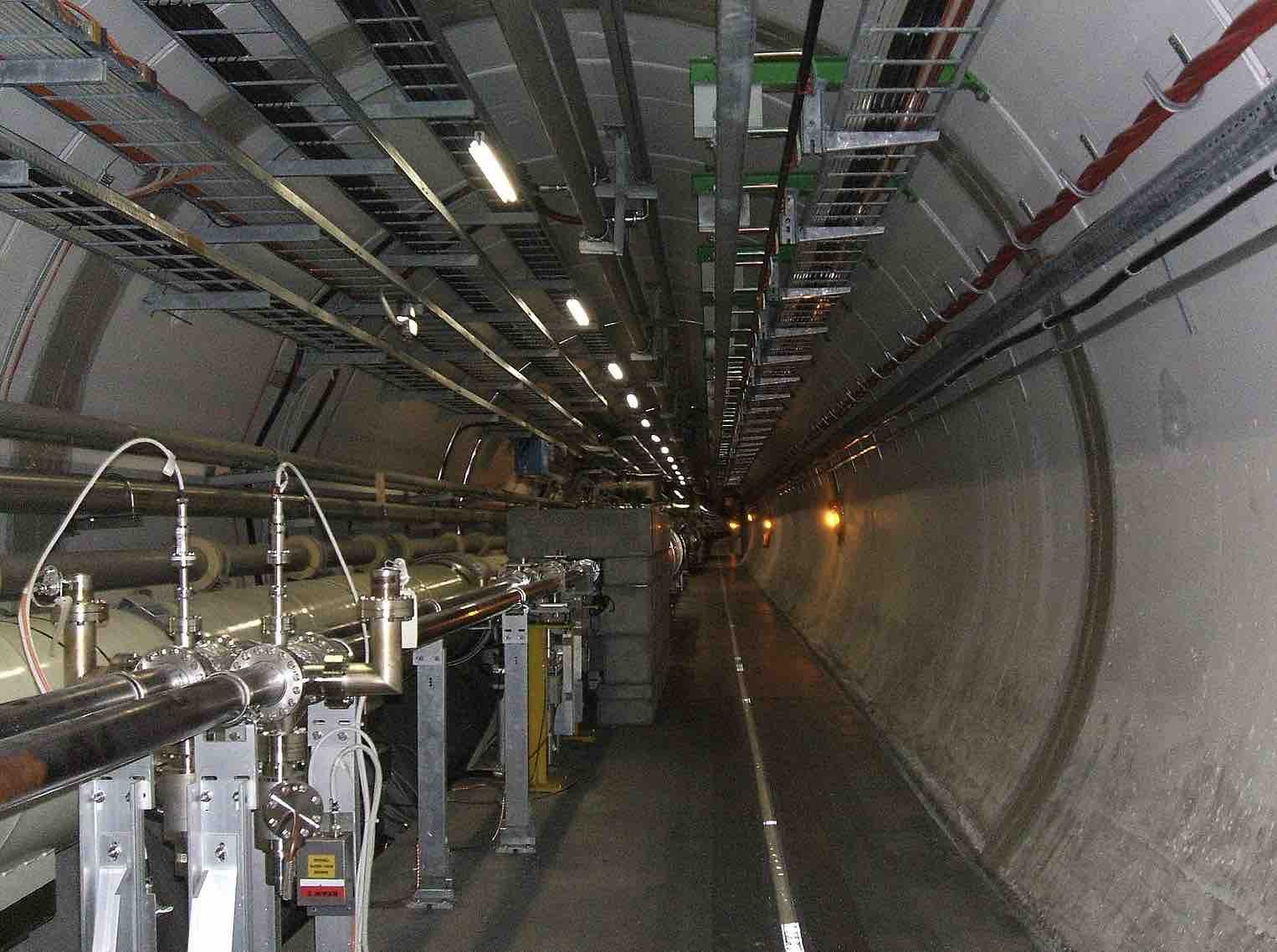
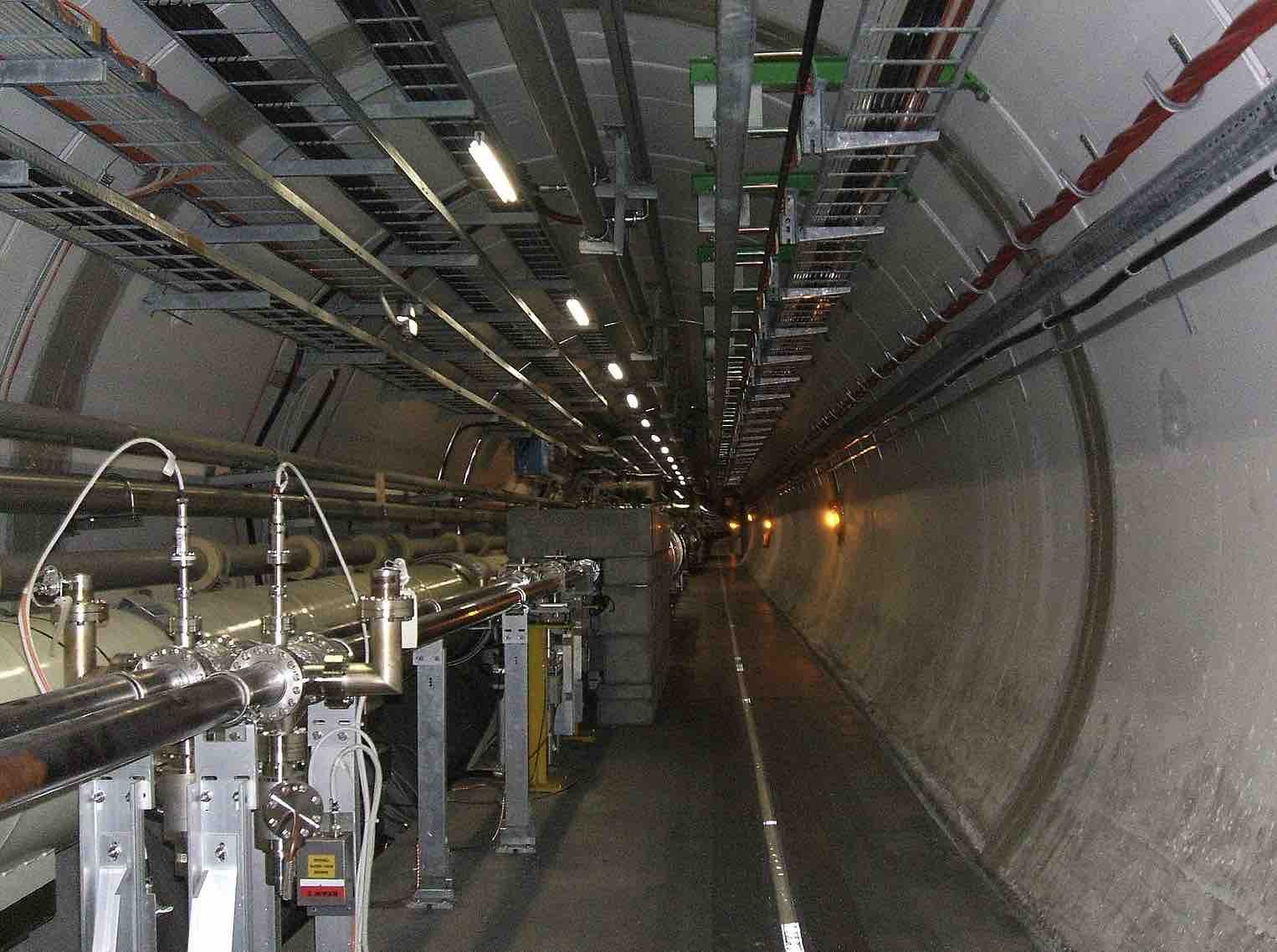
By contrast, analyzing proton-proton collisions within the LHC presents significant difficulties for research, mainly due to the substantial background noise that is generated during proton-proton collisions. Additionally, protons are not elementary particles like their electron cousins. Despite some of the challenges inherent to such studies, experiments such as ATLAS, CMS, and LHCb have still contributed an abundance of new highly precise data.
Nonetheless, in its recent experiment, the CMS collaboration was able to attain precision comparable to electron-positron collisions using protons, using a sample of collisions gathered between 2016 and 2018 at a center-of-mass energy of 13 TeV that represents close to 11,000 million million collisions.
According to a CERN statement, the mixing angle during the experiment was derived from analyses that resulted in what is now recognized as the most precise measurement ever performed using a hadron collider.
Additional details of the recent analysis can be found on CERN’s CMS Physics Analysis Summaries page.
Micah Hanks is the Editor-in-Chief and Co-Founder of The Debrief. He can be reached by email at micah@thedebrief.org. Follow his work at micahhanks.com and on X: @MicahHanks.