Scientists funded by the U.S. Department of Energy (DOE) have shown the ability to compress, or “squeeze,” infrared light by 90%. Previous techniques involving bulk crystals showed some marginal ability to compress the wavelengths of infrared light, but this new approach is many orders of magnitude more effective, opening up a number of possible applications.
“We’ve demonstrated that we can confine infrared light to 10% of its wavelength while maintaining its frequency – meaning that the amount of time that it takes for a wavelength to cycle is the same, but the distance between the peaks of the wave is much closer together,” explained Yin Liu, co-corresponding author of the paper outlining the team’s breakthrough, and an assistant professor of materials science and engineering at North Carolina State University “Bulk crystal techniques confine infrared light to around 97% of its wavelength.”
According to Liu, their process, which uses thin-film oxide membranes instead of crystals, has established “a whole new class of optical materials” that can control light in infrared wavelengths, “which has potential applications in photonics, sensors, and thermal management.”
Transition Metal Perovskite Materials Squeeze Infrared Light
To harness the ability to squeeze infrared light, Liu and colleagues explored the use of a material known as transition metal perovskite membranes. According to the press release announcing the experimental breakthrough, using these types of materials to squeeze infrared light had been theorized but never put to use. “Theoretical papers proposed the idea that transition metal perovskite oxide membranes would allow phonon polaritons to confine infrared light,” Liu says.
To build their own customized transitional metal perovskite membrane, the team used pulsed laser deposition, a process in which a small laser is used to essentially “grow” a crystalline structure from scratch.
In this case, the team used this advanced process to grow a 100-nanometer-thick crystalline membrane of strontium titanate (SrTiO3) in a vacuum chamber. Because they were grown in a vacuum chamber using the pulsed laser, the thin-film materials were of very high quality, meaning they had very few defects.
Once completed, these thin films of material were then removed from the substrates upon which they were grown and transferred to the silicon oxide surface of a silicon substrate material. Now, the researchers only needed the right equipment to test their ability to squeeze infrared light.
Synchrotron Near-Field Spectroscopy at Lawrence Berkeley National Laboratory
According to the team’s research, which is published in the journal Nature Communications, they reached out to the folks who operated the Advanced Light Source of the Lawrence Berkeley National Laboratory. This specialized facility is perfectly equipped to perform synchrotron near-field spectroscopy on the strontium titanate thin films while exposing them to infrared light. If the theory was correct, the films would show the uncanny ability to dramatically compress the wavelengths of infrared light without affecting their frequency.
Numerous experiments were run, and as hoped, the material worked extremely well. The infrared light was not only compressed down to 10% of its wavelength, but the frequency remained unchanged.
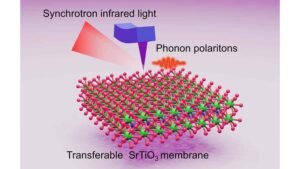
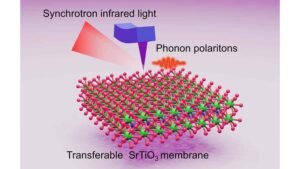
“This behavior was previously only theorized, but we were able to demonstrate it experimentally for the first time through both the way we prepared the thin-film membranes and our novel use of synchrotron near-field spectroscopy,” says Ruijuan Xu, co-lead author of the paper and an assistant professor of materials science and engineering at NC State.
In fact, the process was such a success that all of the infrared light was confined within the substrate. According to Liu, this precision control of infrared light demonstrates that the membranes do indeed confine the light’s individual photons while also keeping them from “extending beyond the surface of the material.”
Applications for Night Vision and High-Performance Computer Chips
Although these materials have so far only been constructed in a lab setting, the researchers behind this process say their method is easy to replicate. They also note that their process creates a material that can be easily integrated into a wide range of substrates, opening up their uses to numerous electronic devices and their subsystems.
“The work is also exciting because the technique we’ve demonstrated for creating these materials means that the thin films can be easily integrated with a wide variety of substrates,” Xu says. “That should make it easy to incorporate the materials into many different types of devices.”
The researchers also say that their material’s unique properties could be used in night vision technologies or other imaging technologies that seek to improve the resolution of images captured using infrared light systems. They see potential applications in high-performance computer chips, which are often challenged to operate in high-temperature environments.
“The thin-film membranes maintain the desired infrared frequency but compress the wavelengths, allowing imaging devices to capture images with greater resolution,” Liu explained. “Imagine being able to design computer chips that could use these materials to shed heat by converting it into infrared light.”
Christopher Plain is a Science Fiction and Fantasy novelist and Head Science Writer at The Debrief. Follow and connect with him on X, learn about his books at plainfiction.com, or email him directly at christopher@thedebrief.org.