Researchers studying the so-called ‘magic angle’ at which two-dimensional graphene becomes a superconductor say the magic part comes from the fact that electrons exist as both particles and waves. This dualistic nature of electrons has been repeatedly demonstrated via the double-slit experiment, and now it appears that it may also help unlock the door to room-temperature superconductors.
Twistronics and Magic Angles
Since researchers first discovered how to arrange carbon atoms into a single layer, essentially creating a material with only two dimensions (which they call graphene), the unique and often times puzzling properties of this material have continued to intrigue and confound. Among the most exciting aspects of graphene is something engineers refer to as a magic angle. In short, if you place two layers of graphene on top of each other and then twist the dual-layered structure to precisely 1.08 degrees, it becomes superconductive. Some researchers refer to the study of this extraordinary property twistronics.
“Today, we understand better even at just the stacking level how these materials behave,” said theoretical physicist and UCR Professor Vivek Aji, in a previous story for The Debrief. “In twisting, you arrive at a series of ‘magic angles’ where certain aspects repeat. Twisting is the future in this line of research.”
Still, even though the exact magic angle at which bilayer graphene becomes a superconductor is now widely known, scientists don’t quite understand how. Now, a team of researchers from The Ohio State University has conducted their own experiments, and their results indicate that the answer may lie with something even more confounding, the fact that electrons can exist as both a particle and a wave.
Quantum Geometry Revealed in the Double Slit Experiment May Hold the Answer
When bilayer graphene is folded to the magic angle, its electrons stop in their tracks. Since conventional superconductors work by high-speed electrons transporting power, this should mean that graphene isn’t only a poor superconductor, it shouldn’t conduct electricity at all. But somehow the opposite happens.
“The conventional theory of superconductivity doesn’t work in this situation,” explained Marc Bockrath, a professor of physics at Ohio State and co-author of the paper outlining the team’s findings.
“Under the conventional theory of superconductivity, electrons moving this slowly should not be able to conduct electricity,” added study co-author Jeanie Lau, also a professor of physics at Ohio State.
“(So), we did a series of experiments to understand the origins of why this material is a superconductor,” said Bockrath.
According to their study, which was published in the journal Nature, Haidong Tian, the first author of the paper and a student in Lau’s research group, used precision care “to obtain a device so close to the magic angle that the electrons were nearly stopped by usual condensed matter physics standards.” However, like previous bilayer graphene, the sample still exhibited superconductivity.
“It is a paradox,” said Lau. “How can electrons which move so slowly conduct electricity at all, let alone superconduct? It is very remarkable.”
Determined to find an explanation, the researchers decided to tap into the growing body of knowledge in quantum geometry, a discipline that attempts to quantify the unusual and sometimes contradictory nature of sub-atomic particles.
“We can’t use the speed of electrons to explain how the twisted bilayer graphene is working,” said Bockrath. “Instead, we had to use quantum geometry.”
That meant looking into the properties of electrons, and their dualistic nature as both particle and wave, a finding exposed by the now infamous double slit experiment. And because electrons can be waves, that means they have wavefunctions.
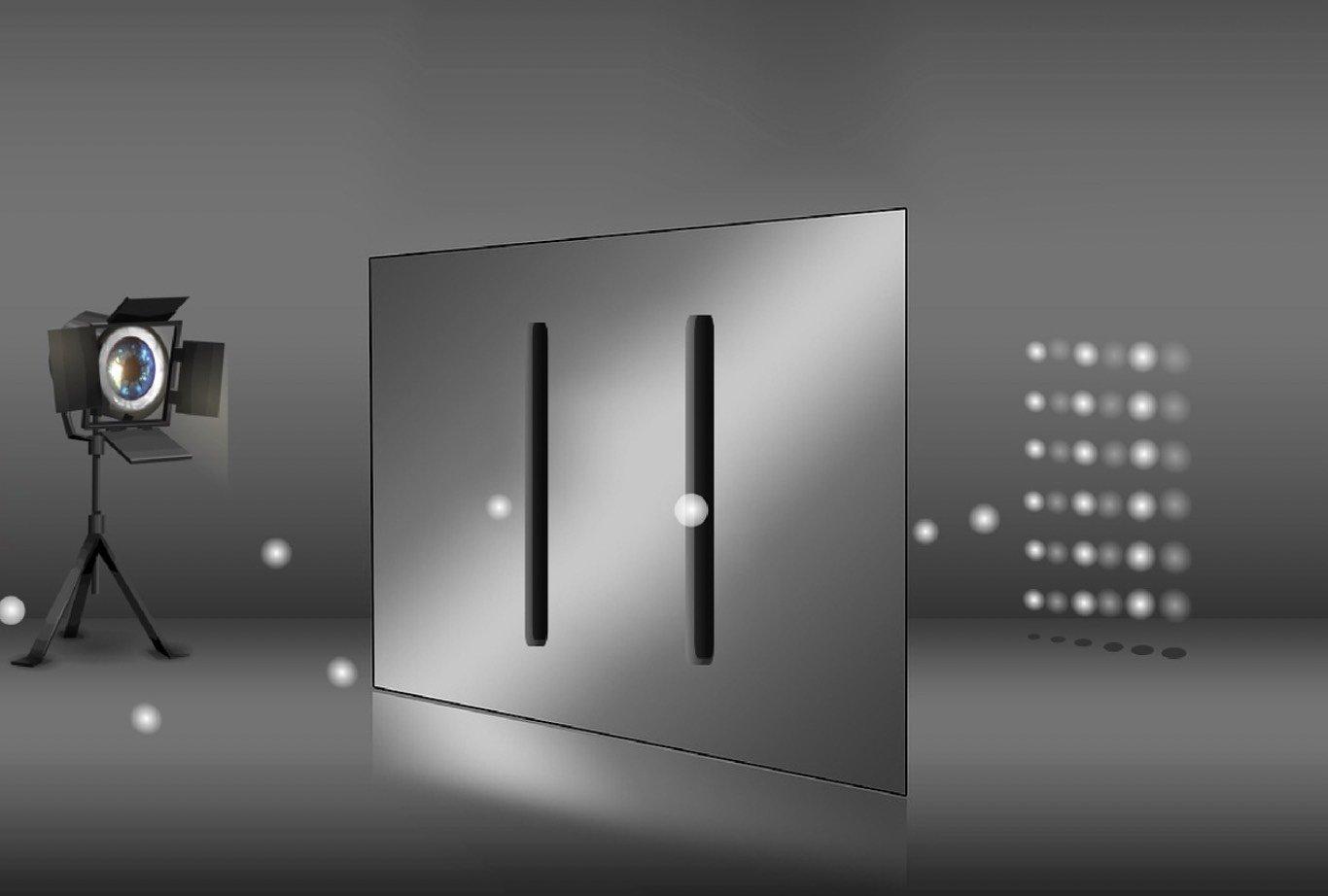
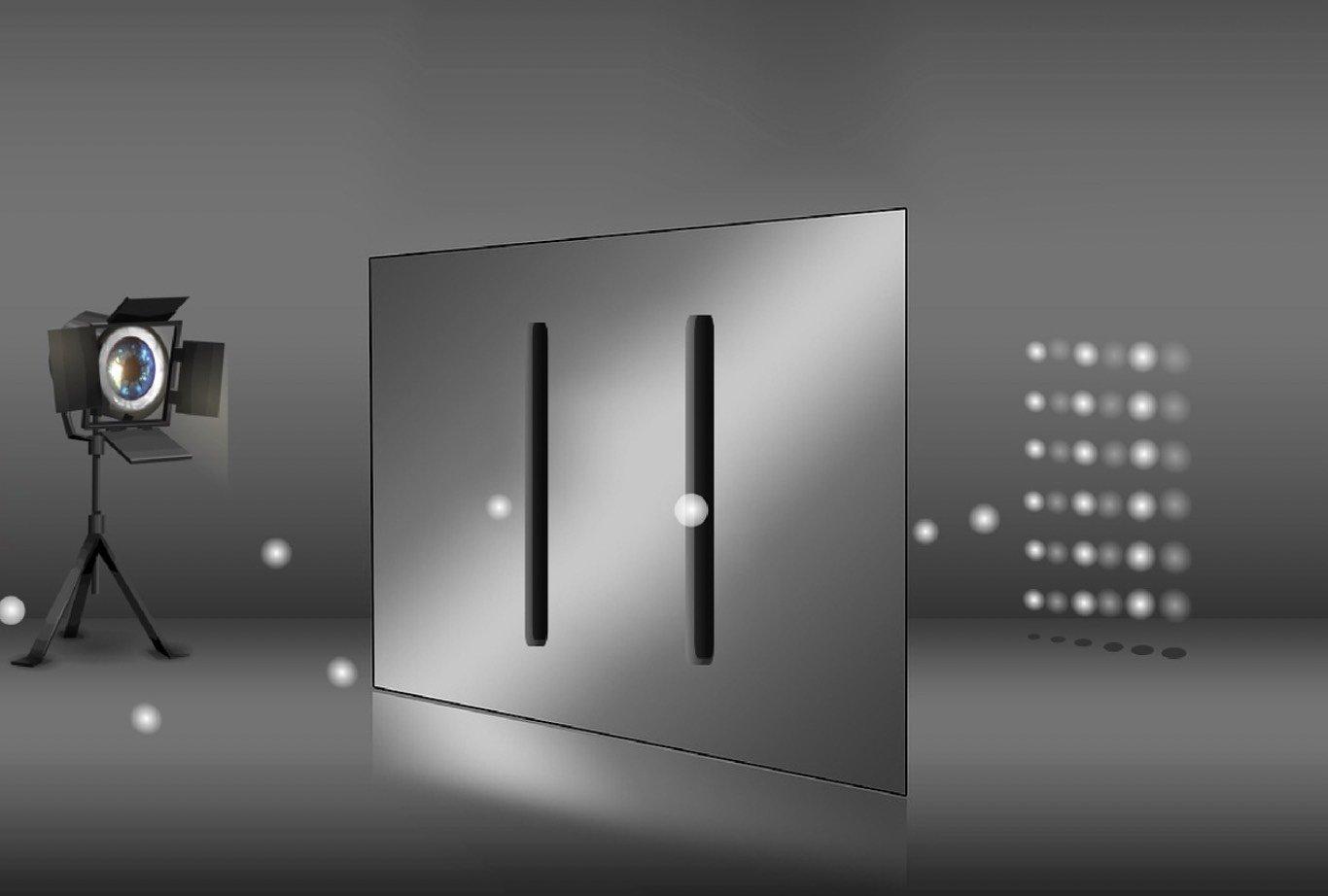
Knowing this, the team theorized that the wave component of electrons is allowing for superconductivity in bilayer graphene where the particle nature of electrons seems like it should prevent superconductivity. It’s a counterintuitive conclusion at best, but one that the Ohio State research team says fits their experiments and the known models of superconductivity perfectly
“The geometry of the quantum wavefunctions in flat bands, together with the interaction between electrons, leads to the flow of electrical current without dissipation in bilayer graphene,” said co-author Mohit Randeria, professor of physics at Ohio State, explaining how this dualistic nature seems to allow magic angle graphene to become a superconductor.
“We found that conventional equations could explain maybe 10% of the superconductivity signal we found,” added Lau. “Our experimental measurements suggest quantum geometry is 90% of what makes this a superconductor.”
The Ultimate Goal is Still Room Temperature Superconductivity
The research team behind these exciting findings notes that the superconductive effects of magic angle bilayer graphene can only be found at extremely low temperatures, a situation that has kept virtually all superconductive materials trapped in the lab instead of in wider use. However, given the insights that this latest set of experiments seems to provide on just how and why ‘magic angle’ graphene can become superconductive, the researchers behind the findings believe it can move the whole endeavor closer to the holy grail of room-temperature superconductors.
“It would have a huge impact on society,” said Bockrath of finding such a room-temperature superconductor. “It is a long way off, but this research is definitely taking us forward in understanding how it could happen.”
Read More: “Twistronics” May Lead to a New Era in Quantum Communication and Night Vision
Christopher Plain is a novelist, comedian, and Head Science Writer at The Debrief. Follow and connect with him on Twitter, learn about his books at plainfiction.com, or email him directly at christopher@thedebrief.org.